Geodesy
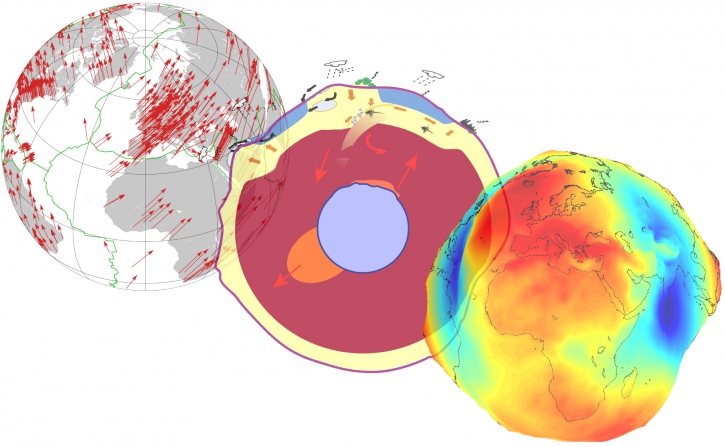
Presentation
Space geodesy and gravity observations are uniquely sensitive to deformations of the Earth under various forcings (e.g. water loads at the surface) or as a result of its internal dynamics, as well as to associated mass redistributions at all depths. This is why they can provide original information on the Earth’s rheology and internal dynamics, while allowing monitoring of the external fluid envelopes.
The exploitation of these observations presents several challenges, on which the Geodesy team is working. It is a question of integrating the information from different sensors in a coherent way to construct the most accurate crustal velocity and displacement fields possible over the long term and over the entire globe, and of separating the different types of superimposed signals and noise in the data. This methodological work goes hand in hand with geophysical modelling of the identified signals, in particular for the study of earth rheology, the seismic cycle and water balances in the outer envelope.
The Geodesy team was created in 2019 by the merger of the Gravimetry and Spatial Geodesy team (IPGP) with the LAREG team (IGN) after the integration of the Institut National de l’Information Géographique et Forestière (IGN) among the supervisors of the UMR IPGP.
The team’s areas of expertise allow it to support the development of new space, airborne and surface sensors in terms of their scientific specifications or the development of dedicated analysis methods.
Several members of the team participate in the coordination and animation of the national thematic pole ForM@Ter in Solid Earth.
Research themes
Mass redistributions within the Earth system (solid Earth, oceans, atmosphere, continental hydrological reservoirs, ice caps) induce variations in the Earth’s gravity field at all time and space scales. Since 2002, these variations have been monitored by the GRACE (2002-2017) and GRACE Follow-On (since 2018) satellite gravity missions with a temporal resolution of 10 days to 1 month, and spatial resolution of 300-400 km. By providing a unique constraint on mass displacements, including at depth, space gravity is indispensable for the study of many geophysical phenomena: monitoring of continental water reserves, mass balance of polar ice caps, causes of sea level variations, post-seismic processes, etc.
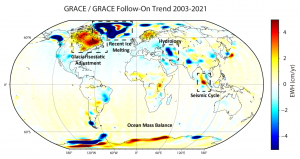
Gravity variations converted into equivalent water depths – Prévost et al., Geophys. J. Int. 219(3), 2034-2055 (2019)
The position and velocity of a point on the Earth’s surface are known thanks to space geodesy measurements, which make it possible to determine the distances between a network of ground stations and a set of artificial satellites orbiting the Earth (including the satellites of the GPS, Galileo, DORIS, etc. systems). Their definition, and the combination of these different types of observations, requires them to be expressed in a reference frame, i.e. a trihedron centred as close as possible to the instantaneous barycentre of the Earth’s masses, associated with a length scale. The precise determination of the Earth’s deformations requires at least as precise a knowledge of the parameters of this reference frame. It is essential for many civil and scientific applications: positioning and navigation, orbitography, sea level, deformations of the Earth (e.g. earthquakes, post-glacial rebound, etc.).
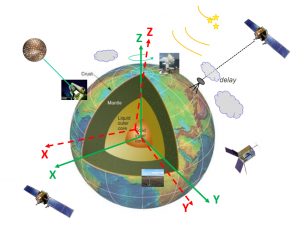
Reference marker of an Earth that deforms at every moment – Credits Z. Altamimi, L. Métivier
The fields of quantum technology and metrology have seen a tremendous growth in the last few decades in time/frequency measurement. With optical atomic clocks that achieve relative frequency uncertainties, Δν/ν, of the order of 10-19 (an error of 1 second per 300 billion years), it is now possible to do chronometric levelling, thought up in 1983, and explore the applications that these instruments offer in geodesy and geophysics.
The idea is based on the use of gravitational spectral shift, a relativistic effect, which predicts that the beat of a clock depends on the speed at which it is moving and the strength of the surrounding gravitational potential. This means that if we compare the beat of two clocks with an uncertainty of 10-18, then it is possible to measure directly between these clocks their difference in gravity potential, ΔW, with a sensitivity of the order of 0.1 m2/s2 or their height difference, ΔH, with centimetre accuracy. This type of measurement is original and unprecedented, as conventional geodetic techniques allow the gravitational potential to be determined indirectly only from conventional gravity and levelling data.
These observables can provide new information on the structures at the origin of the spectral signatures of the gravity field at medium and long wavelengths, and their location at great depths. On the other hand, they can help to better model our altimetric reference surfaces (geoid), characterise the errors in levelling benchmarks and unify vertical reference benchmarks with decimetric – centimetric accuracy.
The ANR ROYMAGE, which started in 2021, attempts to answer these geodetic and geophysical questions.
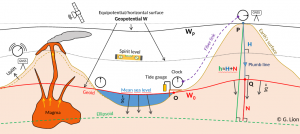
An atomic clock is compared with a reference clock on the topographic surface. Their comparison can be used to determine their mutual difference in height or to detect mass transfer at depth. A clock beats the second faster when moving away from the masses or in the presence of an internal structure that has a lower density than the surrounding medium.
The signal transmitted by space geodetic systems are impacted during their propagation through the atmosphere (ionosphere and troposphere). For radiofrequency techniques (GNSS, DORIS and VLBI), propagation delays due to water vapour in the troposphere have long been the main error source for the precise determination of the vertical component of station position. The improvement of the propagation models used in scientific software now makes it possible to estimate this component with an accuracy of 5 to 10 mm, typically for a 24-hour observation session, except in the case of extreme meteorological events and/or small scale water vapour fluctuations near the station. This calculation is accompanied by a joint estimate of “wet” tropospheric delays (due to water molecules in the atmosphere) with a level of precision and a rate compatible with the needs of weather forecasting models, via assimilation of data within one hour of measurement. The technique is currently evolving to meet the needs of monitoring global change (measurement of the increase in the total water vapour content of the atmosphere under the effect of global warming). New methodological challenges in the field concern the reduction of absolute biases in the estimation of propagation delays and, correlatively, of the vertical component of spatial geodesy techniques, and the detection of breaks in time series due to instrumentation changes of, or environmental changes near, the stations.
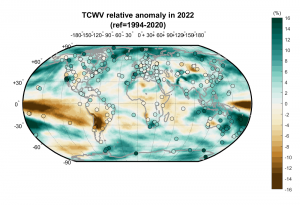
Relative anomaly for the year 2022 of the integrated water vapor content in the atmosphere estimated by GNSS stations (circles) superimposed on the 2D field of the ERA5 reanalysis of the ECWMF.
In detail