The first steps in Earth core weather forecasting
Why has the Earth's magnetic field intensity decreased by 10% between 1840 and the present day? Will the South-Atlantic anomaly in this field, above which satellite equipment suffers the most damage, become even more pronounced in the coming century? When will the next magnetic pole inversion occur? Operational geodynamic modelling aims to answer these questions, and is now providing some initial insights in two articles published in Nature Communications and Geophysical Journal International.
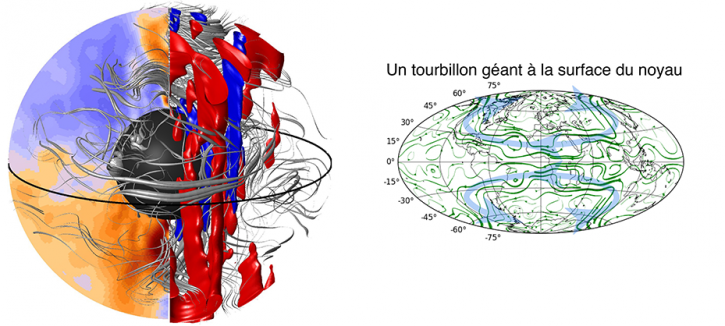
Publication date: 27/01/2016
Press, Research
Related teams :
Geological Fluid Dynamics
Related themes : Earth and Planetary Interiors